Can tech-based carbon removals mitigate climate change?
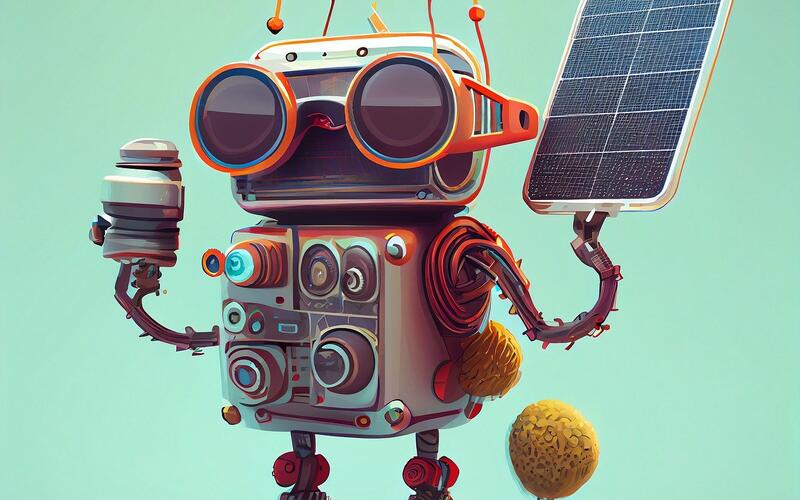
• Desport, L., Gurgel, A., Morris, J., Herzog, H., Chen, Y.-H. H., Selosse, S., & Paltsev, S. (2024). Deploying direct air capture at scale: How close to reality? Energy Economics, 129, 107244. https://doi.org/10.1016/j.eneco.2023.107244
• Fuhrman, J., Bergero, C., Weber, M. et al. Diverse carbon dioxide removal approaches could reduce energy–water–land system impacts. Nat. Clim. Chang. 13, 341–350 (2023). https://doi.org/10.1038/s41558-023-01604-9
Direct air carbon capture and storage (DACCS) — using machines to filter CO2 out of the air and pump that CO2 back into the ground — is one example in a long list of climate mitigation technologies. DACCS is a type of carbon dioxide removal (CDR). This means that it can cancel out carbon emissions from any other source, including those done in the past. While DACCS is a proven technology with several plants either already running or under development, the current scale of operation is minuscule in the context of global greenhouse gas emissions. Some, especially those described as “techno-optimists,” believe that with continued support, DACCS can scale to a massive global industry thousands of times its current size, providing gigatons of carbon dioxide removal and helping to stabilize the climate. Others are more skeptical: they argue that the expense and resource intensity of the DACCS industry will render potentially meaningful differences too little and too late. Accordingly, those wary of the sector say investing in DACCS is a dangerous distraction from genuine decarbonization.
Recent research sheds light on the merits of these two perspectives. One study, “Deploying direct air capture at scale: How close to reality?” published in the Journal of Energy Economics, explores a wide range of possibilities for the evolution of DACCS. Another study, “Diverse carbon dioxide removal approaches could reduce impacts on the energy-water-land system,” published in Nature Climate Change, considers DACCS part of a broader portfolio of potential negative emissions technologies. Both studies use Integrated Assessment Models (IAMs) — models of future scenarios that consider greenhouse gas emissions, technology choices, and resource use across the entire economy — to evaluate the role that DACCS could play in a decarbonized future.
Researchers have been using Integrated Assessment Models to explore future climate change scenarios for decades, helping policymakers understand the interplay between technology changes, greenhouse gas emissions, and the climate system. However, until just a few years ago, carbon dioxide removal technologies were either not included in these models or limited to a single technology. Given the increasingly recognized unavoidable need for harmful emissions to stabilize the climate and the ever-increasing urgency of their deployment with each passing year of largely unabated global emissions, researchers have been working to fill this gap.
Most modeled scenarios start with the premise that we will meet a specific climate goal. They almost always set at a warming level of 1.5 or 2°C above pre-industrial levels in 2100 and then ask, “What combination of technologies would allow us to achieve this outcome the cheapest way?” It’s important to note that the models don’t address how — or if — policies will be implemented to achieve this target. They assume it will be done.
It has already been robustly shown that to limit warming levels to 1.5°C by 2100 — and, increasingly, two °C — we will need both dramatic, rapid emissions reductions and a significant volume of carbon dioxide removal. Both studies’ modeled scenarios follow this pattern: global greenhouse emissions drop precipitously from their current level of ~55 GtCO2e yr-1 (gigatons of CO2 equivalent per year) to well under half that level by 2050. Negative emissions scale up more slowly, growing mainly in the second half of the century to reach levels of about 20 GtCO2 yr-1 by 2100.
The sheer scale of this transformation means that any meaningful technological contribution will also invoke a substantial demand for global resources. Many CDR technologies rely on land directly, whether through growing biomass for energy, spreading rock dust for enhanced weathering (EW), or simply increasing forest cover — and there’s only so much land available. In contrast, DACCS doesn’t use a large land area. Instead, it requires substantial amounts of (net zero carbon) energy to run the machines. This energy could be generated with dispersed renewables. An approach that would demand large amounts of land. Other attempts depend on fossil fuels with carbon capture and storage or involve nuclear, which, while less land-intensive, holds other risks. IAMs allow the measurement and trading-off of these diverse impacts.
Desport et al. trade-off DACCS with only bioenergy with carbon capture and storage (BECCS) and land-use change as other CDR options. This choice matches previous studies, which often only included BECCS as a proxy for all CDR. Without DACCS, they estimate that BECCS alone would use 8.4 million km2 of arable land for crops — about the area we currently use to grow food crops [source].
Fuhrman et al. include a broader range of other CDR technologies, which is the first major study to do so. As well as BECCS, they model spreading rock dust (for enhanced weathering) and biochar on existing agricultural land. While this doesn’t ‘use up’ any land as the same land is also used for growing crops, only a finite area is available. In their scenarios, they consider deployment at the upper limits of the maximum physical potential for both technologies, using all available cropland across the globe and requiring the transport of 13 Gt yr-1 of rock to achieve 5.5 GtCO2 yr-1 of removals in 2100. This level of sequestration is far from guaranteed to be safely possible and is far short of the ~20 GtCO2 yr-1 of total CDR required — implying that BECCS, DACCS, and some other technology must make up the difference.
Therefore, both studies show that not using DACCS means relying on either devoting a vast area of land to growing biocrops or using some other CDR technique that wasn’t included as an option in these models. Given that these are highly uncertain possibilities, this could mean that large-scale DACCS is required — no matter its price and the challenge of deploying at scale. Desport et al. model a scenario with limited land for BECCS, represented by increasing land prices with an increasing fraction used for bioenergy, and find that even if DACCS remains very expensive, it would still need to be deployed at significant levels (a cumulative total of 173 GtCO2 by 2100).
Supposing, optimistically, that one or more of the other non-DACCS CDR options is available at a sufficient scale to provide all required harmful emissions, the question of DACCS’ role in climate mitigation then boils down to how it competes. In the framework of IAMs used by these studies — and, arguably, in the real world — policymakers or removal-credit-purchasers will opt for whichever of the available technologies can remove carbon at the most accessible price.
By making assumptions about the base price for each technology and modeling and tracking the prices of required inputs such as energy, transport, labor, and capital over time, these studies consider plausible future price scenarios for each CDR technique in the context of the broader socioeconomic and technological shifts we expect over the rest of the century.
Fuhrman et al. consider a narrower range of cost forecasts: they use a central scenario with plausible but optimistic prices for DACCS and all other CDR options. Based on this, they find that DACCS delivers a minority, but still significant, level of around 5.5 GtCO2 yr-1 of removals by 2100, or about 16% of the cumulative CDR total. Over the next few decades, DACCS will be entirely outcompeted by cheaper removals from enhanced weathering. Still, as enhanced weathering is fully ‘maxed out,’ DACCS too rapidly scales up after 2060 to meet demand.
Desport et al. fill an essential gap in IAM research by exploring a wide range of base prices for DACCS (between $180 to $1,000/tCO2), combined with a comprehensive set of capture technology and energy supply options, to examine DACCS’ potential based on our full range of uncertainty of its future. They find that at the lower cost estimates, DACCS fully out-competes other CDR options and rapidly scales up starting in 2040 to almost 25 GtCO2 yr-1 by 2100 — comparable in scale to today’s oil industry. On the other hand, at their highest cost estimate and with no limits on land use for BECCS, virtually no DACCS is deployed.
Desport et al. supplement this analysis with a further constraint on price competition. Generally, in global IAMs, the model is set permanently to choose whichever option is cheapest worldwide. This setup only represents the real world if a perfect global market exists. In the case of CDR, this would mean an international market for carbon emissions. Desport et al. consider a scenario with no such global market: only separate regional markets with the world divided into 18 regions. This concept means that each region must achieve net zero individually and cannot rely on purchased carbon credits from the other areas to offset remaining emissions.
Within this context, resources such as land for growing biomass and energy generation may be significantly limited in some regions (and therefore expensive) but plentiful (and thus cheap) in other areas. They find that in this scenario, even with the highest plausible base cost, DACCS remains competitive in some places, resulting in a significant cumulative global deployment of 135 GtCO2, most of which comes from China and India, where land is in high demand for growing food.
Overall, both studies show that under many plausible future scenarios, DACCS is expected to provide a significant and essential role in climate mitigation. At low or moderate price estimates, DACCS could be a competitive option for at least a substantial fraction of total CDR, meaning we’d see a massive global industry scaling up, especially over the later part of the century — potentially rivaling the scale of the current oil industry. It is also possible that other CDR options fully outcompete DACCS, and this industry doesn’t arise if DACCS costs remain high and other options are plentiful and cheap. However, if other CDR options are limited by pressures on land use or other physical constraints, or if there isn’t a fully global emissions trading market, DACCS could still be an essential mitigation tool, even if it is expensive.
While the future is uncertain in many ways — including the possibility that another CDR option not included in these studies will prove safe, scalable, and cheap — this research provides evidence that DACCS could be a critical tool in a future that involves successfully mitigating climate change. This means that adequate policy support for DACCS over the next few decades, expected to influence delivering lower costs and the ability to scale significantly, could be instrumental in achieving this success. However, this situation is only valid under the assumption that we can achieve dramatic emissions reductions and be willing to pay for emissions removals, which is still an open question.